understood that Brian was referring to a a situation that could arise with no change in reactor or mining technology. during the next generation David MacKay tells us, A once-through one-gigawatt nuclear power station uses 162 tons per year of uranium. This calculation is based on the amount of U-235 extracted from uranium, and the burn rate of U-238 in Light Water Reactors. The U-238 burn rate refers to the amount of U-238 in reactor fuel, that is transformed into plutonium in the reactor, and plutonium will fission inside a reactor. Fast Breeder reactors are capable of converting almost all U-238 into plutonium. Some U-238 will fission aster absorbing a neutron so at least in theory fast breeders can convert close to 100% of their uranium into nuclear fuel. MacKay suggests that fast breeder reactors are
Alvin Weinberg had published a visionary essay, "Energy as an Ultimate Raw Material, or Burning the Rocks and Burning the Sea," during 1959 in Physics Today (vol. 12, no. 11, p. 18).
As far back as 1943, Manhatten project scientists including Phil Morrison, Harrison Brown and Alvin Weinberg began to understand the energy implications of nuclear energy. Weinberg later wrote:
Weinberg was not alone. By the 1950's M. King Hubbert was talking about recovery of uranium and thorium from the rocks.
Hubbert reminds us,
Clearly then if there are enormous amounts of recoverable uranium and thorium in so called low grade ores around the world. The USAEC's estimated $500 in 1969 would be $2900. 45 tons of Southern Appalachian coal cost about $2900 during the winter of 2009. One pound of thorium or in a LFTR or uranium in an IFR would produce the energy equivalent of 1400 tons of coal. Thus the AEC's estimate of a 3 billion ton thorium and a 2 billion ton uranium reserve was based on a recovery cost for thorium that was only 3% of the current cost of coal. If the recovery price for thorium at concentrations of no more than 50 PPM would be 3% of the recovery price for coal. An enormous amount of uranium would also be recoverable at highly attractive prices as an adjunct to the recovery of very low grade mineral ore. For example, gamma ray readings form 131 Fort Worth gas wells indicated a uranium concentration of from 10 to 30 ppm in the enormous Barnett Shale formation. Researchers have suggested that the Barnett Shale formation also contains a rich supply of phosphate. Thus the co-recovery of phosphate and uranium from Barnett Shale seems possible, with more than acceptable EROEI (energy return on energy invested) levels. I have not been able to determine the thorium content of Barnett shale. 
MacKay does recognized some uranium resources that can be drawn on, for example the estimated 22, million tons of uranium in mineable phosphate deposits and in phosphate mine tailings, and the 4 or so billion tons of potentially recoverable uranium in sea water. Research has shown that uranium is recoverable from sea water with low costs and very favorable energy return for the amount of energy invested in the recovery. McKay argues that uranium recovery from sea might be possible, and acknowledges
60-times-more-efficientat burning uranium than Light Water Reactors. In fact, in a fast breeder, one ton of uranium is capable of producing 1 GW year of electricity in a fast reactor. Thus fast reactors are 162 times more efficient than light water reactors. This is the first of a number of strange calculation errors, which MacKay makes during his discussion of nuclear power.
MacKay appears to have short-shirked his research on uranium and thorium geology. McKay, a physicists, simply ignores the writings of Alvin Weinberg, Harrison Brown, M. King Hurbert on the the nuclear fuel supply for breeder react0ors
Alvin Weinberg had published a visionary essay, "Energy as an Ultimate Raw Material, or Burning the Rocks and Burning the Sea," during 1959 in Physics Today (vol. 12, no. 11, p. 18).
As far back as 1943, Manhatten project scientists including Phil Morrison, Harrison Brown and Alvin Weinberg began to understand the energy implications of nuclear energy. Weinberg later wrote:
Phil Morrison could hardly contain his excitement as he showed me his calculations. If uranium were burned ion a breeder, the energy released through fission would exceed the amount of energy required to extract the residual 4 ppm of uranium from granitic rock.Morrison's discovery was to have a profound impact on Weinberg for the rest of his long and illustrious career. Harrison Brown, another Manhattan project scientist, also contributed to Weinberg's vision. Brown, along with James Bonner and John Weir wrote "The Next Hundred Years", a book about future resources.
Weinberg was not alone. By the 1950's M. King Hubbert was talking about recovery of uranium and thorium from the rocks.
Hubbert reminds us,
When a U-235 atom is struck by a neutron, it breaks into fragments known as fission products which consist of other atoms near the middle of the table of atomic numbers, and also releases neutrons which strike other U-235 atoms, thereby maintaining a chain reaction. Each fission releases, on the average, 200-million electron volts of heat which, like the heat of combustion of coal or oil, can be used to drive a steam power plant.Then Hubbert points to the magnitude of the Uranium resource:
The objections to the sole use of U-235 are its scarcity and the large amounts of energy required to separate it from U-238. Hence, very great importance attaches to the possibility of converting the fertile materials, U-238 and Th-232, into fissionable materials by means of the breeder reaction. The breeder reaction for U-238 is shown schematically in Figure 26. In this case, the neutrons from the fissioning of U-235 are used to cause a radioactive transformation of U-238 to Pu-239 which is then fissionable. By a similar reaction Th-232 can be converted to U-233 which is also fissionable. It has been experimentally demonstrated that both of these reactions are possible and are capable of producing from the fertile materials more fuel material than is consumed. Thus, in principle, by means of properly developed breeder reactors, it is possible to consume whole uranium and thorium. In the subsequent discussion it will be assumed that complete breeding will have become the standard practice within the comparatively near future.
Now for the energy that is released by the fissioning of a given amount of uranium (or thorium). As indicated in Table 2, the fissioning of 1 gram of U-235 releases 2.28 x 104 kw-hr of heat, which is equivalent to the heat of combustion of 3 tons of coal or of 13 barrels of oil. One pound of U-235 is equivalent to 1400 tons of coal or 6000 barrels of oil. Within narrow limits the same values are valid for U-238 and for thorium.
the so-called "low-grade" ores are the phosphate rocks and the black shales which have uranium contents in the range of 10 to 300 and 10 to 100 grams per metric ton, respectively. Even so, such rocks are equivalent to 90 to 900 tons of coal or 390 to 3900 barrels of oil per metric ton for the phosphates, and to 30 to 300 tons of coal or 130 to 1300 barrels of oil per metric ton of rock, for the black shales. Even granite, as has been pointed out by Harrison Brown (1954) and by Brown and Silver (1955), contains about 13 grams of thorium and 4 grams of uranium per ton, which is equivalent to about 50 tons of coal or 220 barrels of petroleum per metric ton of granite.Although Hubbert had very little to say about the magnatude of Thorium resources, They can only be described as magnificent. WASH-1097 reported the United States Uranium and Thorium resources. Note that while Hubbert said little of thorium, WASH-1097 reported that for five hundred 1969 dollars a pound, the United States Thorium reserve amounted to three billion tons:
What quantity of uranium in rocks of these various types may there be? An indication of the order of magnitude may be obtained by a glance at the map in Figure 28. The Colorado plateau, which is the principal producer of the high-grade ores, has an estimated ultimate reserve of the order of 50>000 to 100,000 metric tons of uranium. The large supplies, however, are to be found in the so-called "low-grade" ores of the phosphate rocks and he black shales. The Phosphoria formation alone, it is estimated from a recent paper by McKelvey and Carswell (1955), contains about ?400 million tons of uranium. Another 0.5 million tons, at least, can be obtained from the phosphate rocks of Florida and the neighboring states.
The Chattanooga shale in Tennessee contains a stratum, the Gassaway member, about 5 meters thick whose average content of uranium is about 70 grams per metric ton (Kerr, 1955). With a density of 2.5 metric tons per cubic meter, this would amount to about 175 grams of uranium per cubic meter, or to 875 grams per square meter for the total thickness of the member. Then for an area of a square mile the uranium content of this member would be 2.3 X 109 grams or 2300 metric tons. This does not sound impressive, and in fact, as compared with contents of the more familiar metallic ores, it is a trifling amount; nevertheless, the energy content of this member per square mile is equivalent to 30 billion barrels of oil, or to five East Texas oil fields. Uranium-rich black shales of Devonian-Mississippian age, which correlate with the Chattanooga, are widespread in the Mid-Continent area as well as in Tennessee and the neighboring states. In addition, the Sharon Springs member of the Pierre shale of Cretaceous age occurring in an extensive area of North and South Dakota east of the Black Hills is also rich in uranium. No attempt has been made to determine the amount of minable uranium which these shales must contain, but since their areal extent amounts to several hundred thousands of square miles, their uranium content would appear to be as much as several hundred million metric tons.
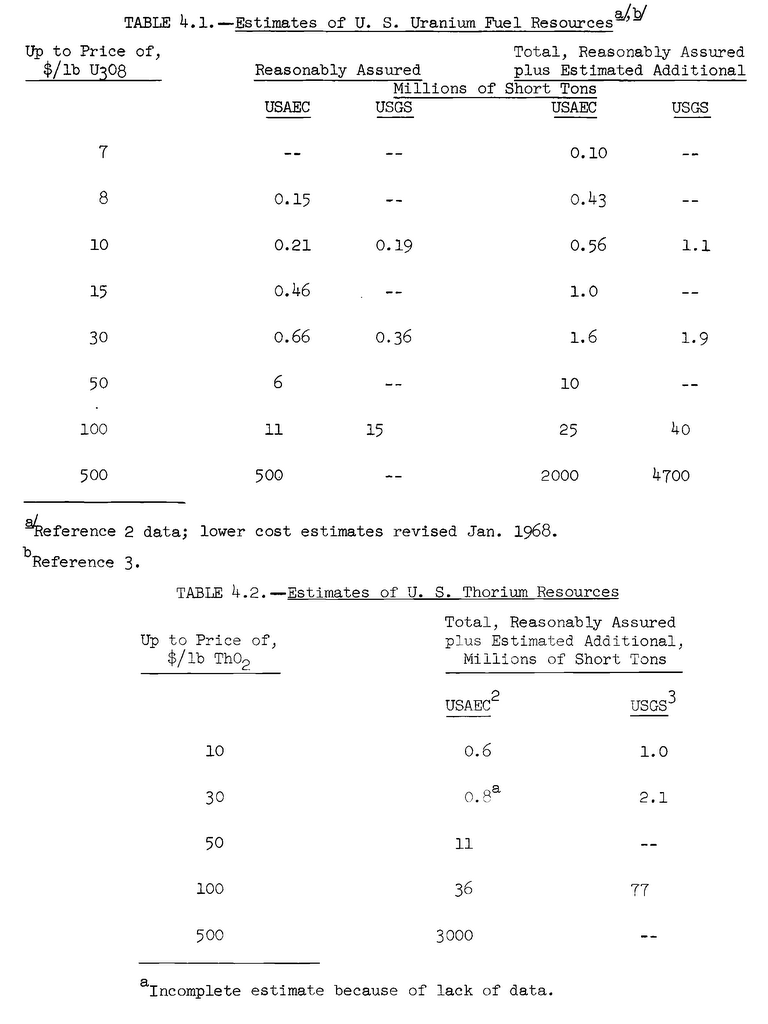

Six test cores drilled in Wetzel County, WV during the 1970's found a consistent presence of 7 or 8 ppm of thorium. Two cores returned about 2.5 ppm of uranium, but two others contained 38 PPM, a fifth contained 58 ppm, and a sixth contained 26 ppm. Radon gas emissions from soil over Marcesllus shale in Ohio and New York states, are consistent with the presence of large ammounts of Uranium and Thorium. The Kentucky Geological Society reported.
Although Hutchins (1971) reported numerous other uranium anomalies in eastern Kentucky, no significant exploration has been conducted to examine the uranium in this part of the state. This deposit fits the classic geologic model for sedimentary uranium deposits, similar to deposits in Colorado and Wyoming. The origin of the Bell Falls uranium is probably related to deeper groundwater in eastern Kentucky, which had been in contact with subsurface black shale, migrated upward, and formed a reducing environment in the coal fragments and conglomerate to deposit the uranium.
Scientists have postulated since 1943 that an enormous amount of energy from supposibly low grade Thorium and Uranium ores, at acceptable EROEI levels. The recovery of large amounts of nuclear raw materials including uranium and thorium appears possible with favorable EROEI. It would also appear that latge amounts of supposably rare minerals such as phosphate are also present along with thorium and uranium in riock formations such as the Barnett shale formation of North Texas. The potential to co-recover these minerals should receive furthr investigation.
Recoverable Uranium and Thorium in shale deposits world wide, have received only limited investigation, but potential resources appear to be enormous. For example, a USGS report states,
David does not list Sweden among the ten top nations with Uranium resources, and indicates a resource of a little over one million tons of uranium for Australia, which MacKay indicated held the worlds largest Uranium reserve. The uranium resources of the Alum Shale of Sweden, although low grade, are enormous. In the Ranstad area of Västergötland, for example, the uranium content of a 3.6-m-thick zone in the upper part of the formation reaches 306 ppm, and concentrations reach 2,000 to 5,000 ppm in small black coal-like lenses of hydrocarbon (kolm) that are scattered through the zone.
The Alum Shale in the Ranstad area underlies about 490 km2, of which the upper member, 8 to 9 m thick, contains an estimated 1.7 million tons of uranium metal (Andersson and others, 1985, their table 4).A report from Aura Energy states
· Aura Energy has made applications for a significant landholding in the major Alum Shale uranium province of Sweden.
· The Alum Shale contains substantial resources of uranium and other metals.
· 77 million pounds of uranium in inferred resources have been identified within 1400 metres of one of Aura's applications.
· Extensive exploration by the Swedish Geological Survey (SGU) indicates the mineralised Alum Shale extends into all of the Aura application areas. Aura therefore interprets that considerable quantities of uranium are present within its applications.
· The Alum Shale has been mined for uranium in both Sweden and Estonia.
MacKay does recognized some uranium resources that can be drawn on, for example the estimated 22, million tons of uranium in mineable phosphate deposits and in phosphate mine tailings, and the 4 or so billion tons of potentially recoverable uranium in sea water. Research has shown that uranium is recoverable from sea water with low costs and very favorable energy return for the amount of energy invested in the recovery. McKay argues that uranium recovery from sea might be possible, and acknowledges
maybe more mineable uranium will be discovered. Indeed, oneIf uranium in sea water were to be recoverable, this should be sufficient to provide all the energy the world needs for a very long time. in addition uranium recovery rates from the sea are sustainable practically forever for as MacKay acknowledges,
paper published in 1980 estimated that the low-grade uranium resource is more than 1000 times greater than the 27 million tons we just assumed.
The uranium in the oceans is being topped up by rivers, which deliverBut something is very long with MacKay's calculations.
uranium at a rate of 32 000 tons per year.
If 10% of this influx were captured, it would provide enough fuel for 20 GW of once-through reactors, or 1200 GW of fast breeder reactors.First the 32,000 tons should be a sustainable recovery rate. If 32,000 tons of uranium are being added to the sea every year, that 32,000 tons should be mineable without diminishing the concentration of uranium in the sea. Thus the 32000 tons is sustainable over the long hall. How much energy is recoverable from this? MacKay calculates 1200 GWs, but as I have noted, MeKay under estimates the amount of electricity that is recoverable from fast breeders. In fact the breeder conversion rate is potentially better than McKay suggested, and even his 10% would produce 3200 GW years of electricity per year. If the sustainable uranium mining rate is from sea water is 32,000 tons, then we have an electrical output of 32,000 GW years. That gives us a sustainable output of 133 kW years per person on the planet, just from the uranium that flows into the sea.
Is this another cargo cult? Even MacKay observes,

a sustainable figure that beats current consumption! – but only with the joint help of two technologies that are respectively scarcely-developed and unfashionable: ocean extraction of uranium, and fast breeder reactors.
There is no great technological hurdle the development of either the fast breeder or to low cost, sustainable uranium extraction from see water, and if these options are unfashionable we have options that might prove more more attractive. Thorium is several times more abundant than uranium, and is mineable with favorable rates of energy returns. The Liquid Fluoride Thorium Reactor (LFTR), a thermal reactor, is capable of breeding thorium, would cost less than fast breeders or light water reactors, is more rapidly scalable, is safer, and would largely solve the problem of nuclear waste. Once the potentials of the LFTR is clearly understood, the nuclear option is likely to grow far more fashionable.
Thus Alvin Weinberg's vision can be realize and has huge implications as he later explained:
In this essay I speculated on the very long-range future-hundreds, even thousands, of years in the future. Where will our energy come from at that distant time when coal, oil, and natural gas have been used up? Solar energy is one obvious inexhaustible source. Another, if it works, could be controlled thermonuclear energy based on deuterium from the sea (thus "Burning the Sea"). My main point, however, was to stress what Phil Morrison and then Harrison Brown had already noticed: that the residual and all but infinite uranium and thorium in granite rocks could be burned with an energy yield larger than the energy required to mine and refine the ore—but only if breeders, which could burn nearly all the fertile material, are used. I spoke of "Burning the Rocks": the breeder, no less than controlled fusion, is an inexhaustible energy system. Up till then we had thought that breeders, burning 50% instead of 2% of the uranium, extended the energy derivable from fission "only" 25-fold. But, because the breeder uses its raw material so efficiently, one can afford to utilize much more expensive—that is, dilute—ores, and these are practically inexhaustible. The breeder indeed will allow humankind to "Burn the Rocks" to achieve inexhaustible energy!In his autobiography Weinberg confessed:
"I became obsessed with the idea that humankind's whole future depended on the breeder. For Society generally to achieve and maintain a standard of living of today's developed countries, depends on the avaliability of relatively cheap, inexhaustible sources of energy."In 1969 as ORNL was, under orders from the USAEC, winding down its brilliantly successful Molten Salt Reactor Experiment, Alvin Weinberg wrote,
The achievement of a cheap, reliable, and safe breeder remains the primary task of the nuclear energy community. (In expressing this view, I suppose I betray a continuing frustration at the slow progress of fusion research, even though the Russian success with the tokamak has quickened the pace.) Actually not much has changed in this regard in 25 years. Even during World War II, many people realized that the breeder was central. It is only now, with burner reactors doing so well, that the world generally has mobilized around the great aim of the breeder.
As all readers of Nuclear Applications & Technology know, the prevailing view holds that the LMFBR (Liquid sodium cooled fast breeder) is the proper path to ubiquitous, permanent energy. It is no secret that I, as well as many of my colleagues at ORNL, have always felt differently. When the idea of the breeder was first suggested in 1943, the rapid and efficient recycle of the partially spent core was regarded as the main problem. Nothing that has happened in the ensuing quarter-century has fundamentally changed this. The successful breeder will be the one that can deal with the spent core most rationally—either by achieving extremely long burnup, or by greatly simplifying the entire recycle step. We at Oak Ridge have always been intrigued by this latter possibility. It explains our long commitment to liquid-fueled reactors-first, the aqueous homogeneous and now, the molten salt. groups working vigorously on molten salts outside Oak Ridge. . . .
. . . indeed, the enthusiasm displayed here is no longer confined to Oak Ridge. There are now several groups working vigorously on molten salts outside Oak Ridge. The enthusiasm of these groups is not confined to MSRE, nor even to the molten-salt breeder. For we now realize that molten-salt reactors comprise an entire spectrum of embodiments that parallels the more conventional solid-fueled systems. Thus molten-salt reactors can be converters as well as breeders; and they can be fueled with either 239Pu or 233U or 235U.
However, we are aware that many difficulties remain, especially before the most advanced embodiment, the Molten-Salt Breeder, becomes a reality. Not all of these difficulties are technical. I have faith that with continued enlightened support of the US Atomic Energy Commission, and with the open-minded, sympathetic attention of the nuclear community . . . the molten-salt reactors will find an important niche in the unfolding nuclear energy enterprise.

8 comments:
Charles
To put the amount of available thorium in perspective for the average person, I love this example:
'LFTR fuel requirements are 1 MT for 1 GW year. Translated into very stark reality: this means that 4 guys, between morning coffee break and their lunch break can dig, with shovels, enough thorium to power a city of one million for one year.'
To be clear I, Brian Wang, said that if we did not change the nuclear reactor technology and did not change the uranium mining methods and still tried to scale up nuclear power to provide 33% of all world electrical power for 50 years. Then the world would start running into uranium supply problems. This was to argue that projections out to 2060 are braindead when they assume that no technology that is not at least 5 years old with a price track record be used in projections out to 2060. Of course technology is changing (new breeders being added and built 2010-2020 in India, russia and china) and new pebble bed reactors. Plus there is new mining methods coming. There will be deeper burn and there will be more power added.
Brian, I am in agreement with you, and perhaps i did not make it clear enough that I was not addressing my commented to you. Reading your email triggered the thinking that lead to this post, but the post was never directed toward you. We are in agreement, and I understood that.
The Japanese have one technology for extracting Uranium from seawater and are working on another, the first being more expensive than mining. I'll have to look up the articles tomorrow.
It's also worth noting that seawater can only hold so much Uranium in solution, the excess has been precipitating out for, well, billions of years. Extracting at a higher rate than the 32,000 tons per year will result in some of that precipiation going back into solution.
How inconvienant that Thorium isn't water soluable.
Uranium mining takes not only energy (mostly oil based)but also causes pollution and disturbs habitat. For this reason less than 100ppm of uranium does not mean an ore. We just have to move to fast reactors and to thorium fuel for sustained nuclear power.
Sodium cooled fast reactors have faced hazards at many places and a safer coolant is called for. Reprocessing also has to be made more economical with a focus on power production away from old weapons plutonium technologies.
liquid salts may be a good alternative coolant as also possibly less fire prone liquid metals. LFTR may be one of the good long term target nuclear technologies but right now there is a lot of U238 lying around in depleted uranium and spent nuclear fuel stores. There is also an urgent requirement of reducing the spent fuel storage volumes demands. Perhaps uranium fueled fast reactors are what is wanted in the next century or so. Russia and India are building such reactors but sodium coolant has to be replaced.
jagdish in situ uranium and thorium mining is not that energy intensive, and energy recovery rates are favorable for thorium even at crustal levels. I am not a big fan of fast reactors. You over estimate the time it would take to develop the LFTR. It is more a matter of money than time. You can either put 100 scientist and engineers to work for 50 years, or put 1000 scientists and engineers to work for 5 years to get what you want.
Next Big Future's summary articles on extraction of Uranium from seawater:
Two proposals (November 2007) at that time the Japanese adsorbent technique would have cost $220 per kilo, at that time prices of mined Uranium were in the $150-$190 range. Not that much more expensive.
Japan's seaweed Uranium extracting plans (August 2008, you've linked to this one before)
Follow up (September 2009).
UK University researchers have develop a technique for the recovery of Uranium from mine waste water (September 2009) the cost would be $140 per kilo Uranium recovered, and it's an environmental clean up technique.
Speaking of clean up, Sparton Resources had a pilot scale demo of a technique to leach Uranium from coal fly ash.
Thorium is a problem by-product in rare earth mining. If a use can be found for it - this would improve the economics of rare earth mines and solve a big environmental problem at the mines.
Post a Comment